Research
Overview:
The research in our lab is based on optical microresonators. These chip-based, micro/millimeter scale photonic devices are able to confine light for up to several hundreds of nanoseconds, effectively compressing several tens of meters of the light beam into microscale structures. This results in high circulating optical power inside the resonator, which makes the optical microresonator a popular platform for studying new physics and photonics applications.
​
In our group, we are particularly interested in studying microresonator-based optical frequency combs (microcombs). Microcombs use Kerr nonlinearity to convert a single wavelength, continuous wave pump laser into hundreds of laser lines with equally spaced frequencies (called frequency comb). Microcombs leverage integrated photonics to miniaturize the Nobel prize-winning comb technology, which could revolutionize the instrumentation in spectroscopy, metrology, navigation, and astronomy.

Three silica microresonators on a silicon chip.
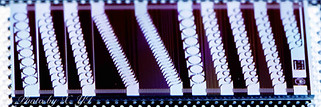
Integrated Silicon Nitride microresonators.
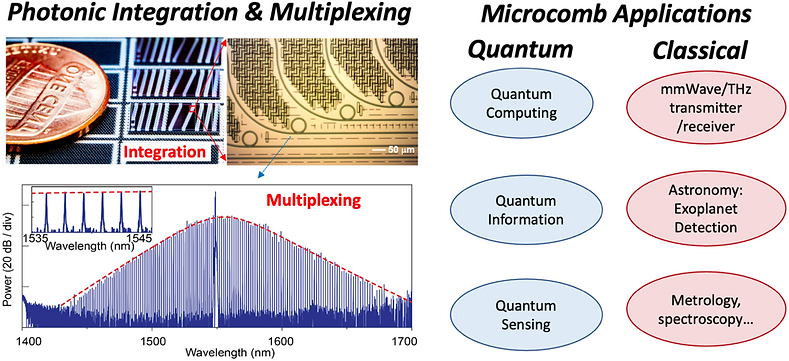
The core of our research is to leverage integration and multiplexing of photonics for various classical and quantum applications. The top left shows the integrated photonic chips in our lab, where hundreds of micro-ring resonators and waveguides are integrated on a millimeter-scale chip. The integration increases the density of optic components by many orders of magnitude and enables mass production. The bottom left shows the frequency/wavelength multiplexing enabled by each micro-ring resonator through the formation of a microresonator-based optical frequency comb (microcomb). Hundreds of laser wavelengths are activated in a single ring-resonator device. The right panel lists the applications for our photonic platforms.
Quantum microcombs
Squeezing and quantum entanglement have been heavily pursued in the past few decades for their wide applications in quantum computing, communications, and sensing. For quantum technology to become a reality, it must undergo an evolution similar in kind to that of the first Bell Labs transistor into massive-scale modern integrated circuits. One of the most promising avenues to mimic such a transformative trajectory is the marriage of integrated photonics with quantum optics.
​
Our group is pursuing an integrated solution by using on-chip optical frequency combs. We recently pushed integrated microcombs into the deterministic quantum regime, where a single microresonator created many pairs of unconditional entangled comb modes (link). These quantum modes can serve as the building blocks for continuous-variable universal quantum computing, frequency-multiplexed quantum communications, and entangled-enhanced sensing.
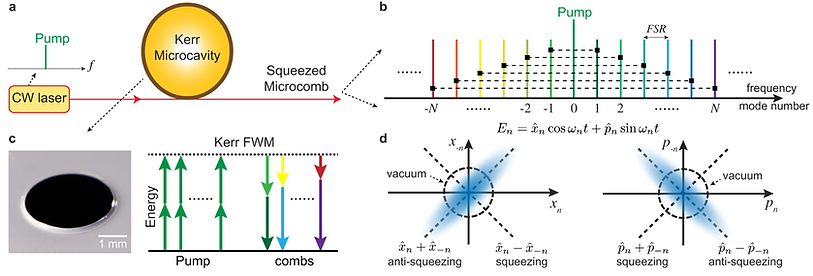
Squeezed quantum microcombs. (a) Illustration of a Kerr microcavity pumped by a continuous-wave (cw) laser. (b) The Kerr nonlinearity creates unconditional EPR entanglement of the optical quadrature fields between frequency modes n and −n. (c) Image of the silica microresonator on a silicon chip and the photon conversion process in the microcavity. (d) Wave-function illustration of two-mode squeezing (EPR entanglement).
In the News

Quantum Microcombs: Scalability is one of the central challenges in practical universal quantum computing. Frequency combs can be used to scale up the number of quantum modes through multiplexing. In this work, we explored the deterministic quantum regime of microcombs, in which unconditional entanglements among hundreds of equidistant frequency modes can serve as critical ingredients to scalable universal quantum computing and quantum networking.
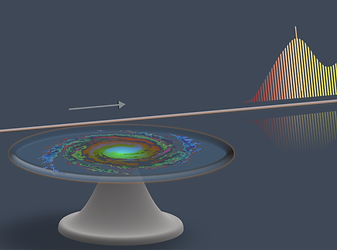
Two octave-spanning microcombs: The spectral span is a critical metric for optical frequency combs. In this work, we report the broadest microcomb, whose spectrum ranges from visible to infrared wavelengths. To output such broad optical spectral from the microresonator to an optical fiber, the microresonator is slightly deformed to allow chaotic motion in the resonator to move the photons from the resonator to the optical fiber.
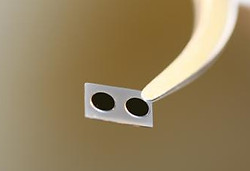
Stokes Soliton: Discovery of a new type of optical soliton wave that travels in the wake of other soliton waves, feeding off of the energy of the other wave. Despite decades of study, this is the first soliton that behaves in a dependent—almost parasitic—manner.
(Photo credit: Qifan Yang, Dr. Ki Youl Yang, Caltech)
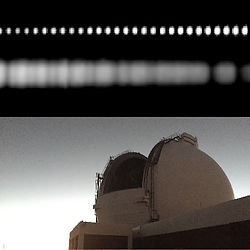
Astrocomb for searching explonets: We built a laser frequency comb to assist in measuring the stellar wabbling caused by its planets. The velocity of the stars that are thousands of light years away from us, can be measured down to 30 cm/s accuracies. The system was tested in the NASA IRTF telescope and W.M. Keck Observatory, Hawaii.
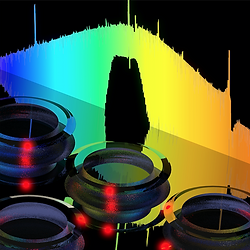
Soliton microcombs on a chip:
A silica microresonator converts a single wavelength pump laser into thousands of equal distance mode-locked laser lines. Numerous applications could benefit from this soliton frequency comb, including optical clock, super GPS, astronomical calibration and etc. (photo credit: Qifan Yang, Caltech)
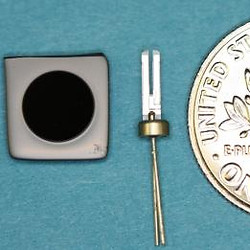
Electro-optic frequency divider.
Using a breadboard-sized frequency comb system, we stabilize microwave signals in the range of gigahertz, or billions of cycles per second—using a pair of laser beams as the reference on a chip. This new technology was referred as electro-optical frequency division. (photo credit: Dr. Jiang Li)